- How do NVD's work?
Night visions let you see in the
dark! So there is a small amount of light (photons) which
went trough the lens of the objective. After the photons are
entering the lens there will got trough a photocatode in the
image intensifier tube (IIT) which converts the photons into
electrons. After that the electrons went trough the MCP (
Micro channel plate). This Part of the night vision tube
does what the name is saying... it multiplies the electrons
what results in a much stronger electron-signal what
amplifying the image what we can see. After the huge amount
of electrons went into the phosphor screen at the
end of the image intensifier tube (IIT) there will be
converted into photons! After all this processes we are able
to see the typical green or white image with our eyes when
we look into a night vision device.
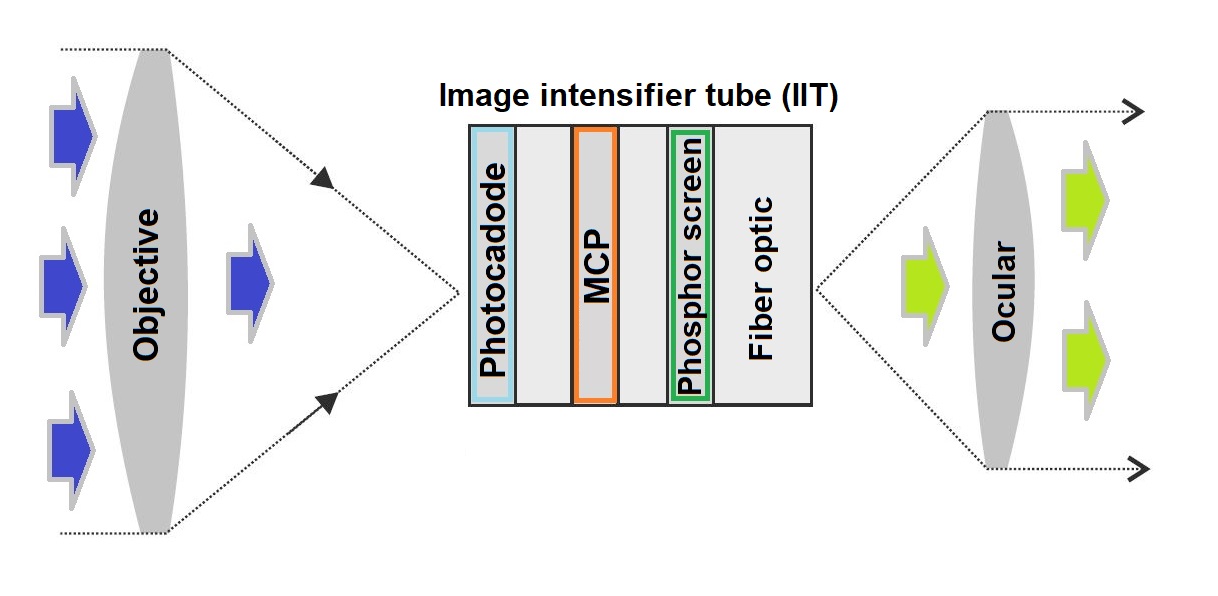
-What tubes are we using?
Photonis ECHO (Commercial grade)
Res: min. 57 lp/mm
SNR: min. 24 Sens: N/A
FOM: min. 1600 – max 2000 Photonis
Echo tubes with wide spectrum bandwidth. They see what others do not see.
Echo tubes allow for more spots, but at a great value and performance. -But
even with some spots there will be no really disadvantages for the normal
end-user, especially in motion spots are not really disturbing.
Photonis
ECHO+ (Commercial grade)
Res: min. 57 lp/mm
SNR: min. 26 Sens: N/A FOM: min. 2000 Photonis Echo+ tubes with wide spectrum bandwidth. They see what others do not see. Echo+ tubes allow for more spots, but at a great value and performance. Compared to regular Echo tubes these have a min 2000 FOM.
Photonis 4G (Milspec grade)
Mil spec grade tubes from Photonis N/A FOM: min. 1800 Photonis 4G tubes with wide spectrum bandwidth and a better performance in lower / no light conditions. They see what others do not see. 4G tubes are allowed to have less spots than ECHO or ECHO+ tubes, but for their performance there are more expensive Compared to regular Echo tubes.
-Green vs. White Phosphor
One of the most essential parts of an IIT is its phosphor screen. It is what you see and what converts the electrons back to photons.
Typically, the phosphor is deposited on the back of the fiber optic and then covered with a layer of aluminum. The aluminum layer increases the light efficiency up to almost 100% by reflecting the light and reducing the electrical charge retained.
There is a multitude of phosphor types that are usually referred with with a P-number i.e. P-22. Four parameters are the most critical ones for evaluating a phosphor screens performance and suitability: Conversion factor, light spectrum, decay time and resolution.
Conversion factor
Refers to conversion rate of
electrons vs photons. So how many photons are emitted by one
electron hitting the screen.
A high conversion rate does not
necessarily mean the eye will perceive a bright image as
that depends entirely on the emitted light’s wave lengths.
Light spectrum
The emitted light spectrum of the
screen i.e. 475nm-600nm for P43.
Decay time
The time the screen needs to go
back to a low brightness after being activated. It has to be
multiple times shorter than the eyes temporal inertia. It is
comparable to the refresh rate of a TV screen or a light
bulb.
Resolution
This is depending on the size of
the average size of particles that are the screen. Bigger
particles would be more efficient but would eventually
create a literally grainy image. Normally this is kept at
about twice the resolution of the final system.
As a fifth indicator serves the luminous efficiency. This is not a fixed number but rather describes how well a phosphor screen produces light within the visible light spectrum or in other words how well the light output of the screen fits the to the human eye.
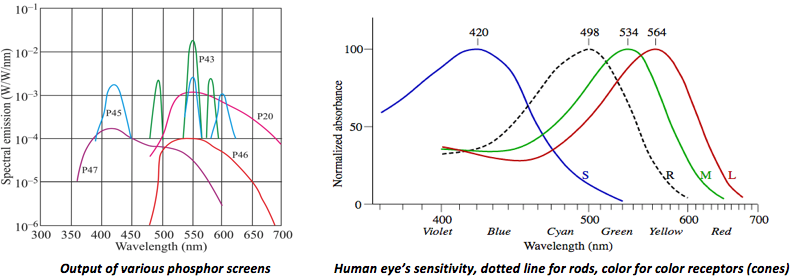
P43 (yellow-green)
Pro:
·
High luminous efficiency, almost the same as the human eye
for the color green
·
Human eye is most sensitive to contrast with green colors
·
Low decay time (1.2 ms to 10%)
·
More yellow-green image compared to older P22 (is not
produced anymore),and less eye fatigue in comparison
·
Much higher conversion rate and light emissions compared to
P45
Con:
·
Faster eye fatigue compared to P45
·
Narrower bandwidth which uses basically just one type of
receptors (cones) in the eye where P45 uses most of the bandwidth
P45 (white)
Pro:
·
White light feels more natural in low light environments and
therefor causing less eye fatigue and
having a noticeably perceived brighter image
·
As recently introduced into (broader) production, most of
these tubes use state of the art technology
·
Low decay time (1.5ms to 10%)
·
White light allows to use the rod cells in the eye which are
most sensitive in low light which
can
create a brighter perceived image
Con:
·
Lower luminous efficiency than P43/P22 which can produce a
darker perceived image
·
Hardly any eye pieces match the bandwidth of P45 which can
cause distortion on the image (chromatic aberration) and hard to design for the wide
bandwidth
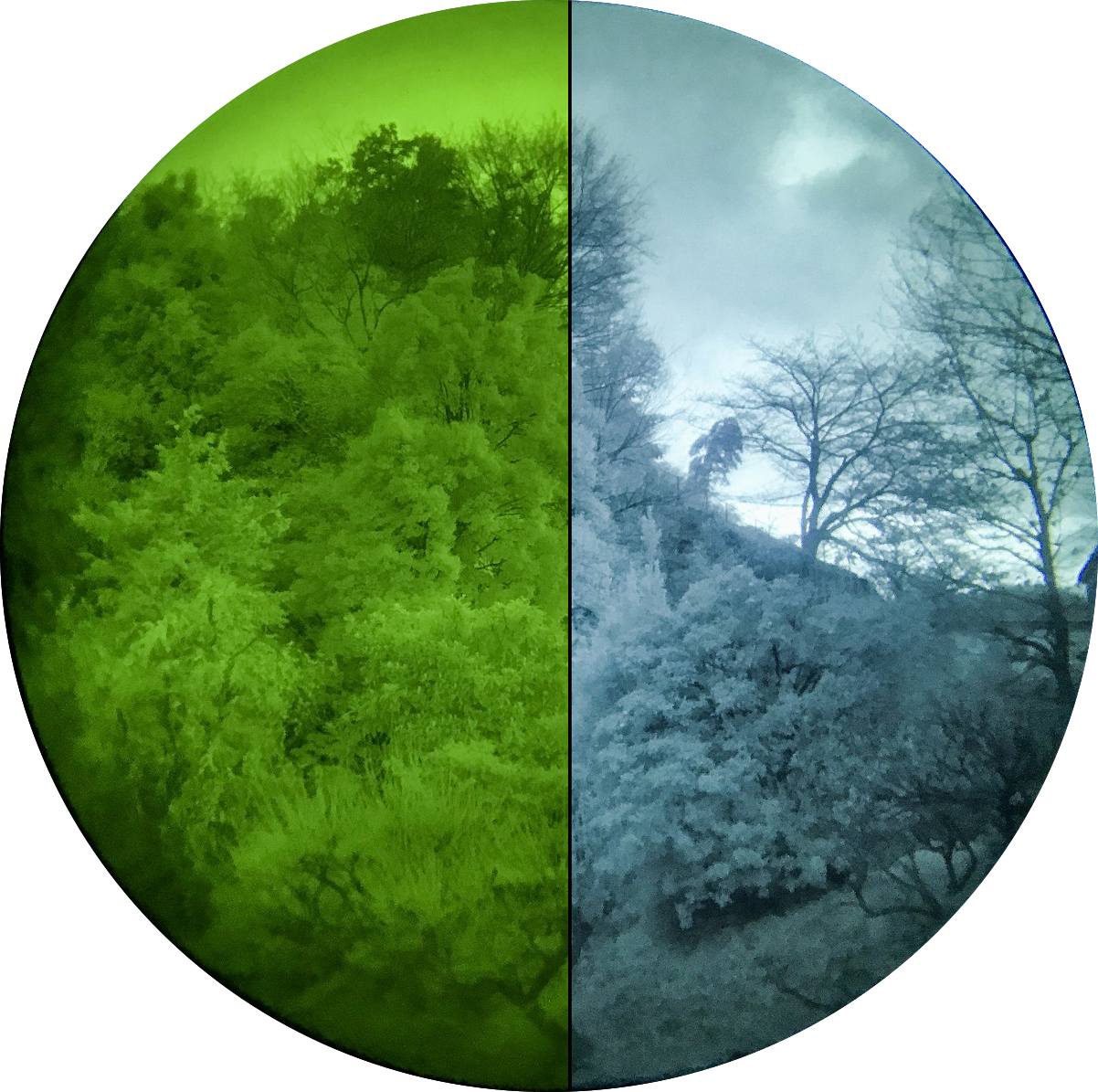
Summary
There are strong arguments between
green and white phosphors and
both have their up and downsides. A noticeable trend from
green to white is
visible in the market, especially the US market and since
P45 tubes are now
certified for aviation this will increase.
As the broader introduction of P45 tubes is fairly recent to
this date, the performance and technology is absolute state
of the art like filmless or 4G IITs which enhances the
performance compared to older P43 or P22 (P22 older green
phosphor) tubes.
As everyone is different and their eyes are different, you
cannot say that P45
or P43 is better as it rather depends on the users and what
they can work
better with. Our recommendation is to try both in a
comparable environment and
decide afterwards.
-Spots
Why are people worried about spots? Of corse it is nicer to have a spotless tube... but spots are cosmetic blemishes in the image intensifier tube which are normal and come from the manufacuring process. We are testing our tubes and document every tube which we are building in our devices. Spots aren`t effect the operation or the reliability of the night vision device. And even in a dynamic scenario where the most people use the devices there will be no distrubing you as a end user.
-The Generations
Generation 0
Early snipers used image converters (sniper-scopes) that required an additional infrared light source to illuminate the scenery. Known as Gen 0, these image converters evolved from RCA's image converter tube developed in the mid-1930s for use in televisions. The Gen 0 image converter used an S-1 photo-cathode, a high-voltage electron acceleration electrostatic field and a phosphor screen. The S-1 cathode (AgOCs) did not have as much quantum efficiency as the cathodes used today, it heavily relied on the IR light used to illuminate the surrounding.
The
process by which the image was intensified was quite simple in
this generation.
It was more a transformation then an intensification,
transforming the
invisible IR light in to visible light. The reflected IR
illuminator light
entered the tube and the photo-cathode converted the light to
electrons.
Electronic elements focused these electrons through a
cone-shaped anode and
accelerated them using high voltage so they hit the phosphor
screen with greater
energy, creating a visible image. This did not produce much gain
and caused
distortion in the image. Also, tube life was not very good by
today's
standards.
Generation 1
The starlight scope, developed during the early 1960s and used during the Vietnam War, was made using Gen 1 image-intensifier tubes. In this scope, three image-intensifier tubes were connected in series, making the unit larger and heavier than today's night-vision goggles. This early generation produced a clear center image with a distorted periphery. The use of multiple tubes connected in series allowed for much greater overall light gain as the output of the first tube was amplified by the second and the second by the third. Due to the simple power supply design, the image was subject to instances of blooming momentary image washout due to an overload in the intensifier tube caused by bright light sources.
The
primary difference between Gen 1 and Gen 0 was the more
sophisticated chemical
process employed to create the photo-cathode. The S-20 cathode,
a multi-alkali
antimonide process, enhanced the sensitivity as well as the
spectral response.
However, Gen 1 did have some of the same drawbacks of image
distortion and
decreased tube life as seen with Gen 0. Tubes built with Gen 0
and Gen 1 technologies
are commonly found in many of today's civilian low budget night
vision viewers.
Generation 2
In the late 1960s, Gen 2 technology brought a breakthrough in night vision with the development of the microchannel plate (MCP). Additionally, the photocathode was further refined to the S-25 cathode and produced much higher photo response.
It was the introduction of the MCP that made Gen 2 unique. The MCP begins with two dissimilar pieces of glass. A large tube of solid glass (core) is placed within a tubular sleeve of glass (clad). The two glasses are then heated together and stretched to form a very small diameter glass fiber. The fibers are ultimately compressed together to form a bundle of glass fibers called a boule. The boule is then sliced at an angle to obtain thin discs. Further chemical processing removes only the core glass, thus creating the channels within the MCP. During the tube operation, the electrons travel into the channels and, as they strike the channel walls, they produce secondary electron emissions which create several hundred electrons, multiplying with each strike.
The
other advancement with Gen 2 was the reduction in overall size
and weight of
both the tube module and the power supply. It allowed for
compact head mounted
night vision devices.
Generation 3
Developed in the mid-1970s and placed into production during the 1980s, Gen 3 was an advance in photocathode technology. Gen 3 tubes use gallium arsenide (GaAs) photocathodes. This increases the tube's sensitivity dramatically and particularly in the near-IR. The increased sensitivity improved system performance under low-light conditions.
However, the highly reactive GaAs photocathode could be easily degraded by the inherent chemical interactions that take place within a tube under normal operation. Most of the chemical reactions take place within the MCP due to the electron interactions with the walls of the MCP channels. Thus, to overcome the degrading effects of the photocathode, a thin metal-oxide coating was added to the input side of the MCP. This coating, more commonly known as an ion barrier film, not only prevented premature degradation of the photocathode but also enhanced the tube life by many times that of the Gen 2 tubes. Today's Gen 3 tubes have a lifetime of at least 15.000h.
Gen 2 and Gen 3 tube manufacturers have made continuous improvements through the years to increase the signal-to-noise ratio within each respective technology. Continuous improvements have been made within MCP manufacturing so as to improve the overall resolution also.
There has been considerable effort expended in developing a Gen 3 tube without the ion barrier film. The effort proved successful, but the manufacturing costs were to high compare to the performance improvements. For a brief period of time, the Gen 3 tube without the ion barrier film was termed Gen 4. This terminology, however, was rescinded shortly after it was announced, though some resellers of night-vision tubes still use the nomenclature. Today L3 is the only company manufacturing these Filmless tubes. L3 calls them infinity tubes.
Continuous developments
One area that has contributed to the improvements is the advancement of the miniature high-voltage power supply. Early developments with the power supply included protection circuits to automatically control the output brightness of the tube under changing input light conditions. These effects, known as automatic brightness control (ABC) and bright source protection (BSP), were directed at protecting both the image tube from highlight exposure and the user's eyes from excessive brightness. The ABC automatically reduces voltage to the microchannel plate to keep the image intensifier output brightness within optimal limits and to protect the tube. This effect can be seen during rapid changes from low-light to high-light conditions when the image gets brighter and then quickly returns to a consistent level. The BSP reduces voltage to the photocathode rather than the microchannel plate. The BSP protects the image tube from damage and enhances its lifetime. The scene resolution can be degraded under high-light conditions. Advancements in miniaturized power supplies include the addition of Autogating circuits. These circuits control the way the photocathode is operated under changing input light conditions. Autogating allows the image tube to be used under higher input lighting with much less degradation of the image quality. Autogating turns off the photocathode voltage for brief periods of time, the effect is not visible to the human eye. The cathode voltage is constantly oscillating, but the image appears as if it were continuous. The Autogating circuit reduces the time the voltage is on during each oscillation but keeps the peak voltage level up. By controlling the application of voltage in this manner, the resolution quality remains high. In effect, the Autogating feature tricks the device into thinking it is always in a low-light environment, which is the optimal environment for maximum efficiency and clarity for the image-intensifier tube. While the most obvious effect of Autogating for the user may be improved resolution in high-light conditions, its original purpose was to help extend the lifetime of the tube, a benefit which is most realized with thin-film or filmless tubes. As opposed to the gradual lifetime decay seen in tubes without an ion barrier film, the Gen 3 tube with gating and the use of a thin film improves tube life and performance far more than any other image-intensifier technology. Typical reliability is well in excess of 15,000 hours without noticeable degradation. This change in durability is a significant accomplishment when considering the much shorter lifetimes of Gen 0, Gen 1 and Gen 2 tubes.
-What is state of the Art?
The development of Gen 3 IITs started end of the 1970
and the first contract for Gen 3 IITs (the OMNI I
contract) was signed in 1981.
There are several differences between the Gen 3 and Gen
2+ technology. Main difference is that Gen 3 uses GaAs photocathodes
instead of the multialkali photocathodes used in Gen 2+.
Their conversion-rate is much higher then any of the Gen
2+ tubes. But they have one drawback, due to the nature
of GaAs, they deteriorate much faster and have a
lifespan of only about 200h. So they added a thin
oxidation layer on to it, limiting its conversion-rate
but extending its lifespan to more then 15.000h. This
film layer usually cuts the conversion-rate in half. On
a side note, the conversion rate is also given in most
datasheets and is called the Luminous Sensitivity
measured at withe light (2856°K) and the unit is uA/lm.
(uA being the electrons and lm the light, lumens, so how
many electrons do you get per Lumen) We will go deeper
in to the numbers and how to evaluate a datasheet in
another Sundays post.
The very first Gen 3 IIT was developed in Germany mid 70's. The US was quick to acquire the technology and further develop it. ITT and Litton became the leading manufacturers. Then in Russia, Katod started manufacturing Gen 3 IITs. Today in Germany, the country where it all started, Harder Digital is producing tubes with great performance.
Then there is Photonis www.photonis.com , perfecting the Gen 2+ multialkali technology with astonishing results. Tubes where one is unable to tell a difference compared to a good gen 3 tube. They do have some very distinct advantages, such as excellent highlight performance, a very small halo and shock resistant up to 500G. These tubes are widely used in Europe, both military and civilian applications. The XD-4 and XR5 being mostly used by military and the Supergen line is the civilian version, tubes that did not meet one or more of the military requirements. Mythical Gen 4, yes they do exist, there was a short period where they where officially called Gen 4. Today they are called infinity tubes, manufactured by L3. In the late 90's Litton managed to alter the GaAs photocathode in a way so that one didn't need a oxidation film to protect it from deteriorating to fast. This meant a much better light to electrons conversion rate resulting in outstanding lowlight performance. However, they where expensive to manufacture and very shock sensitive, but they are still even today seen as the nonplus ultra of all IITs.
-Glossary of terms
What does
Automatic Brightness Control (ABC) mean
This allows the tube to
protect itself from overexposure. It keeps the image
within a certain
brightness range with upper limit, meaning there is a
limit to how bright a
tube can get to prevent damage.
Autogated PSU
This is nothing
else then PWM but for image intensifier tubes. Offering
the tube better
protection and preventing resolution loss in high light
condition.
Black Spots
Almost every tube has
them, bigger or smaller. These are dust-particles
trapped in the tube during
the manufacturing process and are almost impossible to
avoid.
Bright Spots
This
is a defective MCP, meaning electrons are being released
even if they
shouldn't. This is a common phenomenon in
well used older tubes. Their
visibility can vary depending on the light exposure
conditions.
Biocular
Using a single image
intensifier tube with both eyes.
Binocular
Viewing a scene through
two image intensifiers, i.e. one channel per eye.
Blooming
Loss in visibility due
to a bright light source. Common in Gen 0 and Gen 1.
Chicken Wire
A pattern
of dark thin lines in the field of view either
throughout the image area or in
parts of the image area. Under the worst-case condition,
these lines will form
hexagonal or square wave-shape lines. This is due to the
structural bundles of
with either the MCP or the
fiber optic.
Diopter
The
unit of measure used to define eye correction or the
refractive power of a
lens. Usually, adjustments to an optical eyepiece
accommodate for differences
in individual eyesight.
Distortion
There
are two types of distortion found in night vision
systems. One type is caused
by the design of the optics, or image intensifier tube,
and is classical
optical distortion. The other type is associated with
manufacturing flaws in
the fiber optics used in the image intensifier tube.
Optical Distortion: Optical
distortion occurs when the design of the optics causes
straight lines at the
edge of the field of view to curve inward or outward.
This curving of straight
lines at the edge will cause a square grid pattern to
start to look like a
pincushion or barrel. This distortion is the same for
all systems with the same
model number. Good optical design normally makes this
distortion so low that
the typical user will not see the curving of the lines.
Fiber Optics Distortions: Two types of fiber optics distortions are most significant to night vision devices: S-distortion and shear distortion:
S-Distortion: Results from the twisting operation in manufacturing fiber-optic inverters. Usually S- distortion is very small and is difficult to detect with the unaided eye.
Shear Distortion: Can occur in any image tube that uses fiber-optic bundles for the phosphor screen. It appears as a cleavage or dislocation in a straight line viewed in the image area, as though the line were sheared.
Equivalent Background
Illumination (EBI)
This is the amount you
see in an image tube that is turned on but there is no
light at all on the
photocathode; it is affected by temperature where the
warmer night-vision
device, the brighter the background illumination. EBI is
measured in lumens per
square centimetre (lm/cm2) wherein the lower the value
the better. The EBI
level determines the lowest light level at which you can
detect something.
Below this light level, objects will be masked by the
EBI.
Eye Relief
The distance a person’s eyes
must be from the last element of an eyepiece to achieve
the optimal image area.
Field-of-View
The
angle of the visible area you see through your night
vision device.
Figure of Merit (FOM)
Image
Intensification tube specification designation,
calculated on line pair per mm
x signal to noise. (FOM=lp/mm * SNR)
Gain
Also called brightness gain
or luminance gain. This is the number of times a night
vision device amplifies light input. It is usually
measured as tube gain and system gain. Tube gain is
measured as the light output (in fL) divided by the
light input (in fc). This figure is usually expressed in
values of tens of thousands. If tube gain is pushed too
high, the tube will be "noisier" and the signal-to-
noise ration many go down. Military Gen 3 image tubes
operate at gains of between 20,000 and 45,000.
Gallium Arsenide
(GaAs)
The
semiconductor material used in manufacturing the Gen 3
photocathode. GaAs
photocathodes have a very high photosensitivity in the
spectral region of about
450 to 950 nanometers (visible and near-infrared
region).
Highlight Shutoff
An
image intensifier protection feature builds in to the
housing, incorporating a
sensor, microprocessor and circuit breaker. This feature
will turn the system
off during periods of extreme bright light conditions.
Interpupillary
Adjustment
The
distance between the user’s eyes
(pupils) and the adjustment of binocular optics to
adjust for differences in
individuals. Improperly adjusted binoculars will display
a scene that appears
egg- shaped or as a reclining figure-8 and can cause
headache and eyestrain.
Interpupillary
Distance
The
distance between the users pupils (eyeball centers). The
95th percentile of US
military personnel falls within the 55 to 72mm range of
IPD.
IR (Infrared)
Area
outside the visible spectrum that cannot be seen by the
human eye (between 700
nanometers and 1 millimeter). The visible spectrum is
between 400 and 700
nanometers. Image intensifiers operate in the near
infrared spectrum and
thermal devices operate in the far infrared spectrum.
Lp/mm (Line Pairs per Millimeter)
Units used to measure image
intensifier resolution. Typically, the higher the line
pair, the better the image resolution. Generation 3
tubes generally have a range of 64 - 72 lp/mm, although
line pair measurement does not indicate the generation
of the tube. Some Generation 2+ tubes measure 28-38
lp/mm, (Gen 2+ at 54-59 lp/mm typically), while a
Generation 1+ tube may have measure at 40 lp/mm.
MCP
(Microchannel Plate)
A
metal-coated glass disk that multiplies the electrons
produced by the
photocathode. An MCP is found only in Gen 2 or Gen 3
systems. MCPs eliminate
the distortion characteristic of Gen 0 and Gen 1
systems. The number of holes (channels)
in an MCP is a major factor in determining resolution.
Modern MCPs have about
10 million holes or channels compared to the previous
standard of 3.14 million.
Photocathode
The
input surface of an image intensifier tube that absorbs
light energy (photons)
and in turn releases electrical energy (electrons) in
the form of an image. The
type of material used is a distinguishing characteristic
of the different
generations.
Photocathode
Sensitivity
Photocathode sensitivity is a
measure of how well the image intensifier tube converts
light into an electronic signal so it can be amplified.
The measuring units of photocathode sensitivity are
micro- amps/lumen (mA/lm) or microamperes per lumen.
This criterion specifies the number of electrons
released by the Photocathode (PC). PC response is always
measured in isolation with no amplification stage or ion
barrier (film). Therefore, tube data sheets (which
always carry this raw figure) do not reflect the fact
that over 50% of those electrons are lost in the ion
barrier. While for most latest 3rd generation image
intensifiers the photo response is in the 1800 mA/lm
(2000 mA/lm for the latest Omni VI Pinnacle tubes), the
actual number is more like 900 mA/lm.
Resolution?
The
ability of an image intensifier or night vision system
to distinguish between
objects close together. Image intensifier resolution is
measured in line pairs
per millimeter (lp/mm) while system resolution is
measured in cycles per
miliradian. For any particular night vision system, the
image intensifier
resolution will remain constant while the system
resolution can be affected by
altering the objective or eyepiece optics by adding
magnification or relay
lenses. Often the resolution in the same night vision
device is very different
when measured at the centre of the image and at the
periphery of the image.
This is especially important for devices selected for
photograph or video where
the entire image resolution is important. Measured in
line pairs per millimeter
(lp/mm).
Reticle (Reticle
Pattern)
An adjustable aiming
point or pattern (i.e. crosshair) located within an
optical weapon sight.
Signal-to-Noise Ratio (SNR)
A measure of the light
signal reaching the eye divided by the perceived noise
as seen by the eye. A
tube’s SNR determines the low light
resolution of the image tube; therefore, the higher the
SNR, the better the
ability of the tube to resolve objects with good
contrast under low-light
conditions. Because SNR is directly related to the
photocathode’s sensitivity and also accounts for
phosphor efficiency and MCP operating voltage, it is the
best single indicator
of an image intensifier’s
performance.
Scintillation
Also
known as electronic noise. A faint, random, sparkling
effect throughout the
image area. Scintillation is a normal characteristic of
Microchannel plate
image intensifiers and is more pronounced under
low-light-level conditions.
Screen
The
image tube output that produces the viewable image.
Phosphor (P) is used on the
inside surface of the screen to produce the glow, thus
producing the picture.
Different phosphors are used in image intensifier tubes,
depending on
manufacturer and tube generation. P-45 phosphor is used
in the systems offered
in this catalogue.
Stereoscopic Night Vision
When two views or photographs
are taken through one device. One view/photograph
represents the left eye, and the other the right eye.
When the two photographs are viewed in a stereoscopic apparatus, they combine to create a single image with depth and relief. Sometimes this gives two perspectives. However, it is usually not an issue because the object of focus is far enough away for the perspectives to blend into one.
Variable Gain Control
Allows the user to manually
adjust the gain control (basically like a dim
control) in varying light conditions. This
feature sets the PVS-14 apart from other popular
monoculars that do not offer this feature.